Backstory
For an astronomy class called "Life on other worlds," the final
assignment was a research essay worth 25%. I spent about the third quarter of
the semester on this paper and was pretty satisfied with it. However, we had to
hand in outlines and updates to our TA's every few weeks. Up until 2 weeks
before the essay was due, my topic was much more broad than it became. I became very nervous about handing it in becauseI didn't
tell my TA that I was altering my topic. Also, the topic itself is pretty
outrageous...it's unlikely that we will be able to discover it while on Earth.
Nevertheless, I handed it in on-time and awaited the hand-back
date anxiously. When that date came, I was unable to attend (I had
another class to get to). A few days later, there was talk about how difficultly
and unfairly the essay was marked. I think the class average was in the sixties.
However, the professor hinted towards the fact that at least someone got a good
mark. I was beginning to panic.
I finally got my essay back about 3 weeks later...right after I handed in
the final exam. My TA wrote the mark on the inside cover. While I was walking
out of the room, I noticed that I saw a zero through the paper...my heart sank.
I then opened it up, fearing the worst, but what I saw was 100/100 along with
"Great Work!" The only other marks on the entire paper were two grammatical
errors (ie. "is" instead of "it").
That having been said, enjoy the essay. It is a science paper,
but was written in order for any university student to read and understand.
Also, one of the figures at the end is blank for now since I
uploaded the Word file but drew the figure by hand. I'll be sure to scan it
later.
-k
March 19, 2007
ABSTRACT
In the search for extra-terrestrial life, it is commonly assumed that any organisms found will function using the carbon-based biochemistry known on Earth. To justify the possibility for life on other worlds, Metrodorus of Chios said, “It is unnatural in a large field to have only one shaft of wheat and in the infinite universe, only one living world.” By the same argument life could exist based on other elements on other worlds. One such exotic biochemistry could have a hybrid phosphorous-nitrogen based organism using atmospheric nitrogen, fixed by anaerobic bacteria, and phosphorous, found in the soil, to synthesize an energy storing compound analogous to Earth sugars. It may be possible for this type of life to exist on Titan, a moon of Saturn, using radiation from the Sun, coupled with radiation from Saturn, as a sufficient energy supply for conducting a process similar to photosynthesis. Other elements, such as silicon, may also prove to be effective at hosting life on other worlds. Silicon-life seems to be probable given that it is similar to carbon. Based on chemical analysis, the biochemistries discussed allow for effective energy production and stability. However, these biochemistries do not prove to be more effective than Earth biochemistry under current conditions.
Throughout the course of history, people have wondered whether life on Earth is a unique, chance occurrence in the vastness of the Universe. With many modern interstellar explorations in search of life, the results are still inconclusive. Perhaps the goals of these missions are too chauvinistic in searching for Earth-like conditions where flora and fauna may thrive. What if there are other forms of life, foreign to Earth. Many planets and moons are comparatively barren of the nutrients deemed necessary for growth on Earth. However, these planets and moons are not without their own compounds that may be used for an alternative biochemistry. Although these places host very harsh conditions, usually inhospitable to life (14), microbes living in unlikely places on Earth (7) allows for the plausibility for life to exist in these extreme conditions. By understanding that the basis for life stems from the chemistries of elements common to biology as it is known today, new biochemistries can be postulated. This paper proposes a nutrient cycle of a possible nitrogen-phosphorous based world. The possibility for this life to be present on Titan, the largest moon of Saturn, is also discussed.
Many known elements have been suggested as a basis for life. Silicon, an element similar to carbon (13), has been discussed as a possibility due to the similarities it shares with carbon, most specifically, charge. Just as carbon is able to hold a charge of +4, silicon, being in the same period as carbon on the periodic table, is also capable of holding a charge of +4. Silicon is also heavily abundant on rocky planets and moons, including Earth (14). Although silicon has been incorporated into a major group of eukaryotic algae as early as 200 million years ago, silicon is not an effective element for harbouring life under current and known conditions around the solar system (12).
Earth-bound life is known to be carbon based. This means that all discovered life on Earth is made of complex molecules that consist of a carbon backbone bound by other elements, including nitrogen, oxygen, hydrogen, sulphur and phosphorous. Although there has been no evidence of life based on anything other than carbon, the possibility of another element anchoring life cannot be excluded since only a mere fraction of the Universe has been examined.
All life on Earth requires nitrogen for growth since nitrogen is a primary element in biological molecules such as proteins and nucleic acids. Hetrotrophs are organisms that need to consume energy since they are not able to convert it from the Sun like autotrophs, such as plants. Although hetrotrophs are able to consume nitrogen in their diets, autotrophs need to absorb it from their surroundings. Earth’s atmosphere is most abundant in nitrogen gas (N2). N2 is very inert due to the stability of the N-N triple bond. Utilizing a symbiotic relationship with anaerobic bacteria, plants are supplied with nitrogen oxides, such as NO2. Bacteria absorb N2 and oxidize it in a very energetically expensive, though very common, reaction. This is one of the crucial steps in the nitrogen cycle on Earth (Figure 1).
Plants on Earth are able to absorb nitrates/nitrites (NO3-/NO2-) directly through their root systems. However, the abundance of nitrogen in the soil, compared to that of the atmosphere, is sparse. For the plant to effectively absorb enough soil-bound nitrogen to survive, it needs a well-developed and extensive root system, forcing the plant to limit the growth of stems and leaves. A plant that relies on nitrogen from the soil is much smaller and nutrient deficient compared to a plant that absorbs the more abundant atmospheric nitrogen through symbiosis (12). Animals that are using the smaller plant as a food source would not be supplied with enough energy and nutrients. Eventually, with the limitation in nitrogen, all subsequent links in the food-chain die due to malnourishment.
Nitrogen-fixing anaerobic bacteria are used to access the supply of nitrogen in the atmosphere. Although oxidizing N2 is quite costly, the advantages outweigh the costs. By tapping into the vast supply of nitrogen in the atmosphere, the stems and leaves of the plant are able to grow more effectively, allowing better nutrition to higher-order animals feeding on the plant (12). For this reason, the presence of N-fixing bacteria on a foreign world could be possible. By hosting these bacteria, plants and higher species in the food chain, may able to survive.
Although nitrogen is not directly required for photosynthesis, it is an integral nutrient, responsible for maintaining the ecosystem. Many of the structures in a cell where photosynthesis can occur are composed of biological molecules anchored by a nitrogen-ring (12). Without a supply of nitrogen, the plant would not be able to form new cells capable of photosynthesis. Without this ability to supply itself and the upper food-chain with energy, an ecosystem could not be established in any world.
Titan has an atmosphere that is similar to Earth’s in that it has an abundant supply of nitrogen gas (14). Similar to Earth, Titan may host bacteria able to oxidize the nitrogen from the atmosphere into forms that plants may be able to utilize effectively. Since this proposal includes life based on other elements, a new nutrient cycle, similar to the N-cycle on Earth, would be required (Figure 2).
A form of extra-terrestrial life could be based on nitrogen and phosphorous. Phosphorous polymers are mildly stable. When coupled with nitrogen, the phosphorous polymer is much more stable (13). Plants and other autotrophs based on phosphorous and nitrogen would absorb nitrogen, as nitrogen dioxide, from a symbiosis with bacteria capable of oxidizing N2. Phosphorous in the soil would be absorbed by the root system of the plant. An energy storing compound, similar to carbon-sugars, would be formed by the reduction of the nitrogen dioxide, releasing oxygen gas into the atmosphere. A heterotroph would consume the autotroph, ingesting the P-N-sugars. Using inhaled atmospheric oxygen, released by the plant as waste, the heterotroph would metabolize the nitrogen-phosphorous sugar, releasing the bound energy. The heterotroph would release waste as nitrogen gas into the atmosphere and phosphorous oxides into the soil. Decomposing bacteria, similar to those on Earth, could metabolize the phosphorous oxides, releasing free-phosphorous in the soil for the autotrophs to absorb.
Photosynthesis on Titan may be difficult due to Saturn’s distance from the Sun. Titan does not have a magnetic field, allowing for radiation from the Sun and Saturn to bombard it’s surface (14). Since these radiations are energetic, they may be utilized by Titan plants as visible light is utilized by Earth plants. By using radiation from Saturn and the Sun, enough energy may be supplied to allow for a photosynthesis analogue. Earth-bound examples of organisms living under high radiation (7) allow for the possibility that life on Titan, next to the heavily radioactive Saturn, may be possible.
On Earth, organisms known as extremophiles dwell in regions where conditions are not favourable for most species. An example of these conditions includes high levels of ionizing radiation, as well as nuclear radiation. In most cases, the species that live in these extreme environmental conditions tend to be microbes of the domains Archaea and Bacteria. However, studies of radioresistant organisms in Minas Gerais, Brazil, where uranium deposits cause high levels of radiation, have shown the existence of radioresistant eukaryotes such as insects, worms and some plants (15). Although the level of radiation on Titan surpasses the levels here on Earth (14), adaptation and chemical differences may prove to make life possible. These radiations may also be analogous to background radiations on Earth.
Everyday, plants, animals and humans are bombarded with radiation, irrespective of location. Although these levels are not extremely high, they are constant (8). As it is known, radiation can allow for health defects in living organism. As much as 80% of the radiation received by humans comes from natural sources such as soil and rocks, radon, cosmic rays and the human body itself (16). The levels of background radiation vary greatly based on location. Areas at high altitudes are exposed to higher levels of cosmic background radiation, while areas of lower altitudes, but rockier landscapes like the Canadian Shield, are exposed to higher levels of terrestrial radiation. In some populated places in the world, such as Ramsar, Iran, natural background radiation far exceeds the amount of radiation released during the Chernobyl disaster of 1986 (15). The graph below shows the differences in radiation released by natural background sources and man-made methods such as Chernobyl and the nuclear bomb. These levels are also compared to the natural levels of radiation in various locations in the world. People of Ramsar do not experience the effects of radiation poisoning experienced by those of the areas surrounding Chernobyl (17). The oddity is explained by adaptation to the radiation, as well as the fluctuation in radiation levels. The organisms in Ramsar are constantly bombarded by high levels of background radiation; there is no extreme change in rate (15, 17). During and after the Chernobyl meltdown, radiation levels in the surrounding area were doubled (5). It was this sudden increase in radiation that caused the harmful effects seen over the past 20 years. However, constant natural radiation does not seem to pose any severe problems to those that have adapted to it. Given that Saturn and the Sun supply Titan with constant radiation, life may be able to adapt to those high levels.
Silicon is more abundant than carbon on most rocky planets and moons, including the Earth (14). It is thought that in the fledgling years of the Earth, as life was forming, silicon-based life co-existed with carbon-based life. Life on Earth cannot be supported by silicon under the environmental conditions present (13). Silicon, being a larger atom, requires much more energy to form double or triple bonds than the smaller carbon atom does. Multiple bonds of silicon also prove to be far less stable than carbon bonds in that they decompose spontaneously on Earth. Silanes, the silicon equivalent to hydrocarbon alkanes, are very unstable on Earth. On some other planets, the environment is very abundant in sulphuric acid compared to water. Under this condition, silanes may prove to be more stable than alkanes (13), possibly supporting life. Finally, life on Earth is dependant on oxides of carbon, such as carbon dioxide. Carbon dioxide, a gas at temperatures where water is a liquid, is introduced into an ecosystem by dissolving it in water (12). The silicon counterpart to carbon dioxide, silicon dioxide, is a solid at liquid water temperatures and is far less soluble. At higher temperatures, where silicon dioxide may be more soluble in water, the water will have surpassed its boiling point (13), making it difficult to support an ecosystem. If life was able to be supported by another compound, similar to water, silicon may be able to support life more readily. However, silicon has been shown to support life as a secondary essential element (12).
During the Jurassic period, a group of unicellular eukaryote algae known as diatoms evolved. A major distinguishing feature of this group of algae was the cell wall. In normal plant cells, the cell wall is composed mainly of cellulose, a polymer of glucose. In diatoms, the cell wall was composed of silica, also known as silicon dioxide. The cell walls, usually consisting of two asymmetrical sides, gave the algae protection against larger, heterotrophic organisms (12). Since silica is difficult to dissolve in water on Earth, fossils of diatoms allow for a nearly perfect cell wall, nearly 200 million years after death. If a similar anomaly of cell composition formed on a foreign world such as Mars, microscopic fossil records could be easily classified.
The Viking missions of the 1970’s had NASA send robotic rovers to Mars in search for water and evidences of organic matter (9, 10). It was said that if organic matter were to be discovered on Mars, it would indicate that extra-terrestrial life exists. Both rovers successfully landed and conducted numerous experiments on Martian soil, but concluded no evidence of life (9). Recent insight into the mission and experiments used show that life may have existed in the samples tested by the rovers (9). To test for organisms that were possibly laying dormant in desiccation, water was added to soil samples that were supplied with sufficient light for photosynthesis. Using radioactive carbon, it was concluded that there was no life in the sample (10). Where water is the fundamental compound for life on Earth, similar compounds may be more effective on other planets with different conditions. On Mars, due to the high temperatures and high iron and carbon dioxide abundance, peroxide (H2O2), is speculated to have hosted life. Evidence of peroxide has been speculated by the analysis of a meteor thought to be from Mars (10). Scientists reviewing the information from the Viking mission realized that the experiments done by the rovers tested for water-based life. These experiments, though effective in concluding the existence of water-based life, would not allow for resuscitation of any life form that was supported by peroxide; the experiments could have been killing the life they were trying to prove existed (9, 10, 13).
The Viking mission is just one example of the chauvinistic approach to the search for life. In recent years, the increased funding for this search have allowed for projects such as the Cassini-Huygens mission to successfully determine the presence and content of massive hydrocarbon lakes on Titan (18). With more interest and funding into space programs around the world, a better understanding of the bodies that surround the Earth will be obtained. With the increase in knowledge, new and more probable hypotheses can be formulated regarding the ever-lasting search for life.
Although the exact energetic analysis regarding chemical reactions needed for nitrogen-phosphorous life does not equal those on Earth, organisms may have been able to evolve to utilize the slightly lower levels. The energy levels possible using this biochemistry could support life. The existence of extremophiles on Earth proves that life can evolve to exist almost anywhere. Based on our current knowledge of chemistry, organisms based on carbon prove to be the most efficient. However, on worlds where environmental conditions differ from the requirements of carbon biochemistry, life must settle for the less efficient, but equally functioning biochemistry.
Appendix:
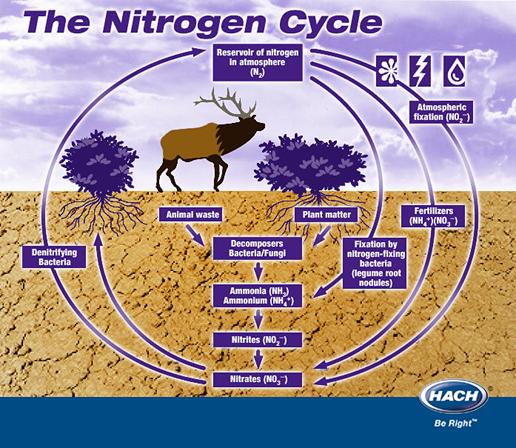
Figure 1 – Nitrogen cycle as it exists on Earth. Available as of March 18, 2007 from: http://www.h2ou.com/h2nitrogencycle.htm.
Figure 2 – Nitrogen-phosphorous cycle as it would appear on Titan.
References and Bibliography:
- Conrad, P.G. and Nealson, N.H. A non-Earthcentric approach to life detection. Astrobiology 2001; 1: 15-24.
- Irwin, L.N. and Schulze-Makuch, D. Assessing the plausibility of life on other worlds. Astrobiology 2001; 2: 143-160.
- Catling, D.C., Glein, C.R., Zahnle, K.J., and McKay, C.P. Why O2 is required by complex life on habitable planets and the concept of planetary "oxygenation time." Astrobiology 2005; 3: 415-438.
- Schulze-Makuch, D. and Irwin, L.N. The prospect of alien life in exotic forms on other worlds. Die Naturwissenschaften 2006; 93: 155-172.
- Papastefanou, C., Manolopoulou, M., Stoulos, S., Ionannidou, A., and Gerasopoulos, E. Background radiation measurements in the lower atmosphere before and after Chernobyl. Journal of Environmental Radioactivity 1999; 42: 87-92.
- Bains, W. Many chemistries could be used to build living systems. Astrobiology 2004; 2: 137-167.
- Cabrol, N.A. Extremes of Life – Abstracts. Astrobiology 2002; 4.
- Choppin, G., Liljenzin, J-O., and Rydberg, J. Radiochemistry and Nuclear Chemistry. Third Edition. Page: 481. Butterworth-Heinemann, 2002.
- Lunine, J.I. Astrobiology: A Multidisciplinary Approach. Pearson Education Inc. as Addison Wesley, San Francisco, California, 2005.
- Lovett, R.A. Mars's peroxide snow would kill any surface life. National Geographic News. August 7, 2006. Available as of March 18, 2007 from: http://news.nationalgeographic.com/news/2006/08/060807-mars-snow.html.
- Adams, F. Origins of Existence: How life emerged in the universe. Pages: 158-190. The Free Press, New York, New York, 2002.
- Raven, P.H., Every, R.F., and Eichhorn, S.E. Biology of Plants. Seventh Edition. Freeman Publishing, 2005.
- McMurry, J.E. Organic Chemistry. Sixth Edition. Brooks and Cole Publishing Co., 2003.
- Kuhn, K.F., and Koupelis, T. In Quest of the Universe. Fourth Edition. Jones and Bartlett Publishers, 2004.
- Kratz, F.L. Radioresistance in natural populations of Drosophila nebulosa from a Brazilian area of high background radiation. Mutant Resistance 1975; 3: 347-355.
- Key, A.W. PHY138Y1 Lecture – Nuclear and Radiation Section – Supplementary Notes 4: The Biological Effects of Radiation. Presented at the University of Toronto on April 9, 2006.
- Masoomi, J.R., Mohammadi, Sh., Amini, M., and Ghiassi-Nejad, M. High background radiation areas of Ramsar in Iran: evaluation of DNA damaged by alkaline single cell gel electrophoresis (SCGE). Journal of Environmental Radioactivity 2006; 2: 176-186.
- Chang, A. Hydrocarbon seas found on Titan. Globe and Mail. March 13, 2007. Available as of March 18, 2007 from: http://www.theglobeandmail.com/servlet/story/RTGAM.20070313.wtitann0313/BNStory/Science/home.
|