Towards a Systematic, Scientific Way for Synthesizing Complex Fluids
Motivation and General Theme
Ordinarily we classify materials as being solid, liquid or gaseous. Complex fluids are mixtures that do not fit into these standard definitions, and sometimes their properties can exceed those of the constituent phases.
Such black boxes are good only for predictions that involve interpolation of existing data and trends, and cannot, in general, be used for improving product performance.

- They must achieve their target function.
- They must be stable over the shelf life of the fabric softener.
- They must have favorable flow properties, and these must not change over the product shelf life.
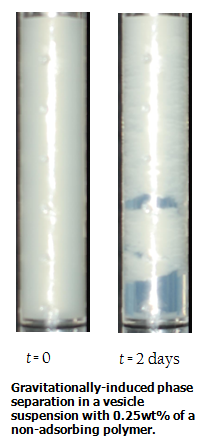
These failures are a consequence of the poor understanding of the empirically-designed preparation procedure.
OUR APPROACH: Break the black box - Understand the system and process fundamentally.
There are several advantages to this approach. First, it allows optimization of process and product function. Products can be manufactured with more efficient utilization of raw materials and utilities. Second, product properties can be tuned precisely to achieve specific functions. Finally, an advantage that is often not recognized is that the knowledge base accumulated from such studies can create opportunities for the design of completely new classes of materials.The general focus of our laboratory is a bottom-up approach for understanding the structure and properties of suspensions of soft particles like drops and cells. Our endeavour is to charactertize suspensions both at the single particle/two particle level and at the multiparticle, concentrated suspension level. The main themes of the projects in our group are presented below, and they all carry the flavor of our general approach.
Projects in our group
1. MEASUREMENT OF NON-HYDRODYNAMIC INTERACTIONS
Non-hydrodynamic interactions determine the equilibrium properties of the suspension, i.e. what the suspension will/should ultimately evolve into. These include electrostatic interactions, van der Waals interactions, etc.These interactions are characterized by the force-separation relationship between two particles. Ordinarily, this is obtained by measuring forces between supported films using devices such as the Surface Forces Apparatus(SFA), Atomic Force Microscope (AFM), etc.
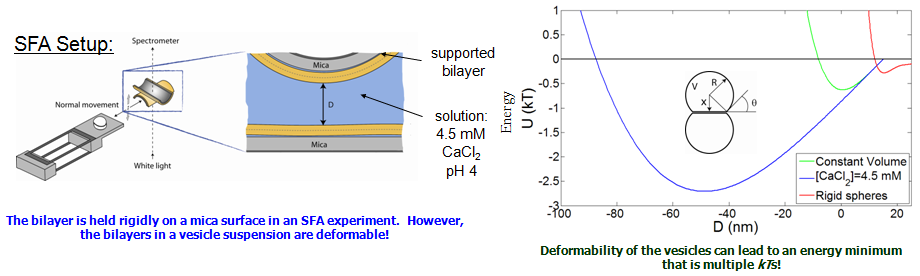

Suraj Borkar and Rajesh Ranjan are the two Ph.D. students working on different aspects of this project. Suraj has developed an inhouse, ray tracing technique to deconvolve RICM (Reflection Interference Contrast Microscopy) interference patterns that resolve separations down to a little below 10 nm. Rajesh is building the force transducer, and will employ the device to study the drainage dynamics of soft microgel particles and vesicles pushed against a flat surface under a constant force..
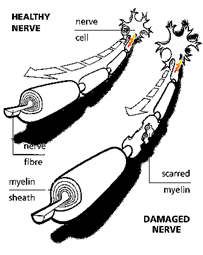
2. MEASUREMENT OF HYDRODYNAMIC INTERACTIONS
Hydrodynamic interactions are non-equilibrium interactions that can change the suspension structure. They also determine the time for non-hydrodynamic interactions to set in. The measurement of these hydrodynamic interactions is currently being accomplished with single and two stagnation point Hele-Shaw microfluidic devices. The single point microfluidic extensional flow device was fabricated by the first MASc graduate student in our laboratory, Ali Hussain Motagamwala. The device is comprised of a diamond shaped microfluidic slot with fluid ports at the corners of the diamond. By altering the flow through the ports using computer-controlled pressure transducers, the stagnation point in the device can be placed at an arbitrary position in the device. This ability allows us to move particles in the slot along arbitrary trajectories.This device is being employed currently to measure interfacial tensions that range from ultra low (microNewtons/m) to high (tens of milliNewtons per meter), to study the breakup of drops, and to characterize solubilities and dissolution rates of drops in the suspending medium. Currently, a Ph.D. student, Sachin Goel is performing these studies for the water-in-bitumen emulsion system in a project funded by Syncrude Canada.
A former MASc graduate student in the group, Dinesh Kumar, has also recently developed for us a six-port two stagnation point microfluidic device, that can control two particles along independent, arbitrary trajectories. A new graduate student, Anas Ettahiri, is employing this device to measure the hydrodynamic drainage times for Hele-Shaw drops for different strain rates, glancing angles, and drop radii.
3. PARTICULATE SUSPENSION FLOWS
It turns out that an initially, spatially uniform suspension of particles may be rendered inhomogeneous by mere application of a flow. This is caused by the phenomenon of cross-streamline migration resulting from particle-fluid and particle-particle interactions, and occurs for suspensions of both rigid and deformable particles. The spatial distribution of the dispersed phase in the suspending medium strongly influences several properties of a suspension such as heat and mass transfer coefficients, conductivity, yield stress (of cured components), etc. Unfortunately, the class of suspensions for which this migration phenomenon is understood in most detail is that of rigid spherical particles in a Newtonian fluid. Needless to say, this represents a small subset of the large variety of possible suspensions. The objective of our work is to develop fundamental understanding of cross-streamline migration phenomenon for flowing suspensions of simple combinations of rigid/deformable, spherical/non-spherical particles in Newtonian/non-Newtonian fluids.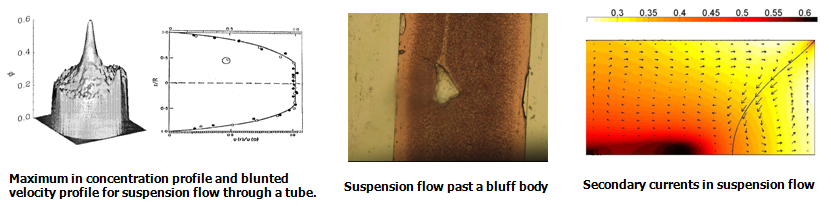
We are currently pursuing the following problems in this area with both simulations and experiment:
- Suspension flow past obstacles in Hele-Shaw geometries
- Solute mass transfer in suspension flows
- Viscous miscible fingering in suspension flows
Apart from these projects, we also have collaborative projects. A Ph.D. student, Yang Li, currently co-supervised by Prof. Eugenia Kumacheva in Chemistry, is studying the translocation of microgels into confinements. The motivation for this work is the development of a fundamental understanding of the diseases, embolic ischemia and pulmonary embolisms. We are also working with Prof. Kumacheva on developing microfluidic platforms to screen a special class of solvents called Switchable solvents; Ghata Nirmal is one of the students working on this project.
TREMENDOUS POTENTIAL FOR IMPACT
The diverse nature of the problem areas and the depth of the questions being investigated in our group places us to signficantly impact a variety of fields, as conveyed by the diversity of the funding organizations supporting our work (see list below).

Fun Stuff
We are also interested in any/all phenomena related to fluid mechanics and/or transport. If there is a problem you wish to work on with us, you are welcome to contact us.
For example, a fun problem that was done not so long ago was the study of the dynamics of a hydrodynamically Janus particle - a particle which exhibits slip on one fraction of its surface and no-slip on the remainder. Such particles perform interesting motions in linear fields. For example, in a simple shear flow, they can exhibit spatially periodic or non-periodic motions (check out the video below).
Funding
We are funded/ have been funded over the past five years by the NSERC Discovery Grant, SynCrude Canada, ACS-PRF, Connaught Award, NSERC Strategic Grant, NSERC Collaborative and Research Development Grant, Canadian Lung Association and Ontario Thoracic Society, North American Mixing Forum Early Career Award, and the Department of Chemical Engineering and Applied Chemistry.
Note: ChE Dept. – Chemical Engineering and Applied Chemistry, UofT – University of Toronto
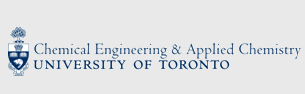
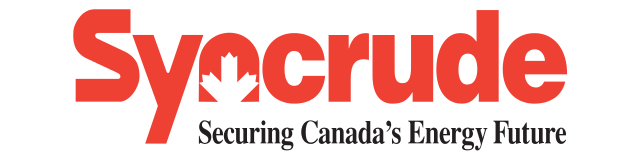

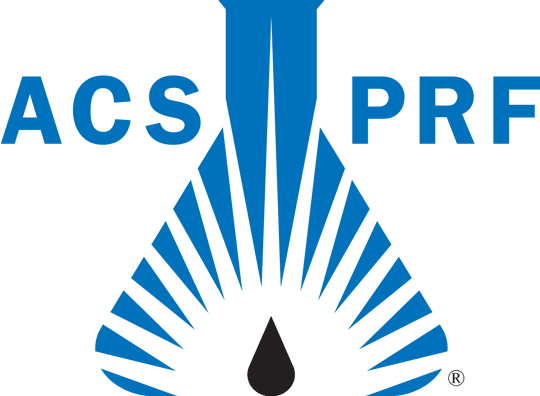
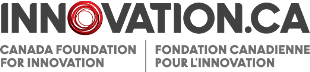
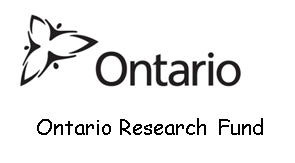


BACK TO TOP