Reviews of current RNA based technologies used in Prion Disease Research
Section Navigator> Scientific Reviews>
                                                                          
> Endogenous ssRNA and shsRNA
                                                                          
> mRNA markers in Prion Diease
                                                                          
> RNA aptamers and Prion Diesase
                                                                          
> RNA interferance and Prion Disease
Therapeutic advances in CJD Prion Disease Using RNA interference
The PrPC gene has long fascinated scientists from the beginning of the identification of Prion disease. From its involvement in the replication and therefore, the pathogenesis of Prion disease, it has been seen as an attractive therapeutic target (1). Since Prion disease is beginning to have a strong impact on the human society seen from the well known Creutzfeldt Jakob Disease (CJD), being able to suppress the PrPC gene expression in CJD is a future mission.
While many drugs have been identified in the suppression of PrPC expression by targeting its’ protein, RNA interference has been regarded as an potential therapeutic method in being able to stably silence PrPC expression by perturbing gene function.
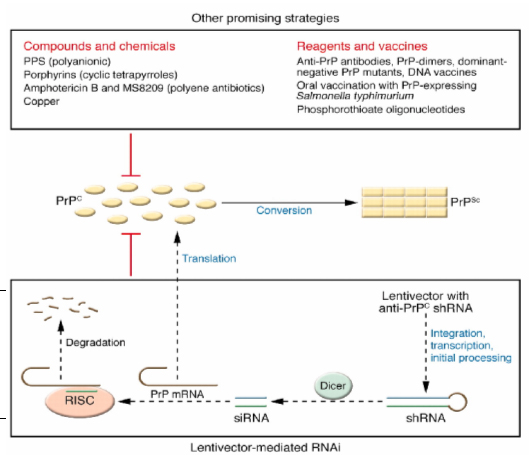
Figure 1: Promising strategies for Prion disease treatment. It is shown that monomeric PrPC converts into multimeric PrPSC. RNA interference and other compounds are shown that are seen to reduce the PrPC levels and therefore have been shown as a therapeutic potential for prion disease. While RNAi involves a complex pathway, the compounds presented have a direct effect. The compounds/chemicals that are most effective and least toxic and that have demonstrated therapeutic effects in animals after intracerebral Prion inoculation are included. (Adapted from Kong, Q. with modifications).
RNA interference is a mechanism in which the presence of small fragments of double stranded RNA (dsRNA) or small interfering RNA (siRNA) whose sequence matches a given gene is able to silence the expression of that gene (2). It has been demonstrated by a study conducted by Daude et al. (3) that siRNA duplexes are able to silence the PrPc gene in scrapie infected neuroblastoma cells. A downfall of the direct transfection by siRNAs is that it only achieves a transient reduction in PrpSC expression (i.e. silencing was not permanent) and indirectly shows that it did not permanently silence PrPC expression. This indicates that in order for PrpC (and consequently PrpSC) to be continuously suppressed siRNA must be continuously added at each round of cell growth. This might be due to the fact that during each round of cell growth, as the cells divided, the concentration of siRNA was reduced in each daughter cell since siRNA was not added at each round. Therefore most of the genes that siRNA targeted were not silenced due to the lower concentrations of siRNA.
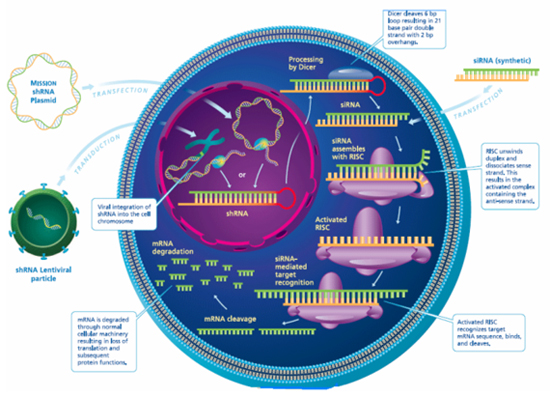
Figure 2: Pathways through which shRNA reduces the expression of PrPC. There are two pathways that may be carried out. One involves the direct transfection of shRNA into a cell. The other is where anti-PrPC shRNA is carried on a lentivector and this is transfected into the targeted cell of interest. Then, it is integrated into the chromosomal DNA and is transcribed. Once transcribed, the anti-PrPC shRNA is released into the cytoplasm, where it is processed by endonuclease Dicer into siRNA. This in turn activates the RNA-induced silencing complex (RISC) to degrade PrP mRNAs, leading to reduced expression of PrPC and consequently improved survival time after Prion infection. (Adapted from Jolliff, K., http://www.dddmag.com)
An efficient delivery system that is now being used is lentiviruses. Lentiviruses are members of the retrovirus family such as HIV that is able to integrate into the genome of not only dividing cells but also non-dividing cells such as neurons, and they have been used extensively in embryonic stem cells and preimplantation embryos (4). Coupling RNA interference with lentiviruses has been shown to be an efficient method at silencing genes. (5) The first study to achieve this, used lentiviral vectors to deliver an shRNA (short hair pin RNA) into goat fibroblasts and target the Prion protein, which was then used to produce a cloned goat fetus. The use of the lentiviruses allowed for the stable suppression of PrPC gene. A down fall of the study was that the suppression was not done in vivo, which might have given different results. It has been shown that dsRNAs when they are applied directly to cells inhibit non-target genes and could activate the innate immune system leading to antiviral responses. (1,6,7) In the case of mammals, it has been shown to activate the mammalian interferon-mediated antiviral response. Therefore using lentivectors as an efficient delivery system not only allows these effects of dsRNAs to be bypassed but also allows for stable suppression of the PrpC gene. This is due to the integration of the dsRNA via lentivectors into the genome which continuously produces siRNA, which are very small and therefore cannot activate any antiviral response.
A study conducted by Pfeifer et al. (4) successfully generated lentivectors from HIV-1 to express PrPC specific shRNAs. In contrast, they used intracranial injections containing these siRNA lentivectors on scrapie infected mice and were able to silence the PrpC gene in vivo. This not only silenced the gene but also led to a stable knockdown of PrpC expression over several rounds of growth. However the injection induced a significant reduction in PrPC expression in the transduced area only. They also generated chimeric scrapie-infected mice by infecting ES cells with this lentivector mediated RNAi and it was found that the ratio of transgenic cells (those infected with lentivector) to the nontransgenic cells was correlated with the level of PrPC knock down in vivo. This showed that these mice had a significantly prolonged survival, which was correlated with the number of cells carrying the lentiviral shRNA vector. This suggests that the more area the lentiviral shRNA vectors are found, there will be an increase in their survival.
One of the weaknesses of the study was that the model organism was the mouse. In comparison to human brain, it has a much smaller brain and its brain is not as complex as the human brain which has a defined blood brain barrier. They put forward this idea by giving us insight that only a limited number of target CNS regions can be injected with lentivectors in humans. They proposed the catheter placement and/or pharmacological opening of the blood brain barrier to deliver lentiviral vectors. This would allow for a more widespread transduction of the CNS in humans.
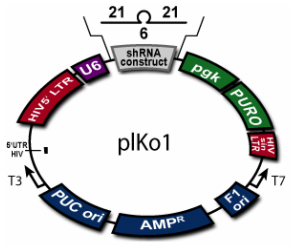
Figure 3: An example of a constructed lentiviral vector plasmid for shRNA expression. (Adapted from BioCat, http://www.biocat.de/cgi-bin/adm/prod.pl?prod=RHS4012-OB)
Finding the right lentivirus vector is critical in terms of safety and efficiency. Sachdeva et al. (8) were able to design chimeric vectors from HIV-1 and HIV-2 and hence were able to construct a suitable lentiviral vector. They accomplished this by incorporating sections of both lentiviruses since HIV-2 is less pathogenic and HIV-1 has higher transduction efficiency. However, lentivectors do have some drawbacks. These include the fact that they require high concentration of virus stocks for their assembly, and their effects may be reduced due to the silencing of many viral components.
Physiological Functions and Gene characterizations
Loss of function of PrPC by RNA interference and knockout experiments was not only seen to suppress the expression of PrPC gene but also has given more insight into its physiological role. Richt et al. (9) conducted a study where they were able to generate PrPC deficient cattle by using knockout vectors to knockout both PrPC alleles in a primary fetal fibroblast line of a male cattle which were then recloned to produce cattle. The cattle appeared healthy in general clinical examination and it was found that PrPC function is not needed for normal animal development. It was also seen that the place of deletion of the PrPC gene is significant in terms of the related physiological outcomes. Deletions of parts of PrPC ORF led to severe effects in mice such as specific cerebellar lesions and ataxia (9). However the complete removal of PrPC as seen in Richt et al (9), led to less detrimental phenotypes. Weise et al. (10) conducted a study on adult PrPC knockout mice in comparison with wild type mice to see whether PrPC has neuroprotective properties. This was done by subjecting these mice to transient or permanent focal cerebral ischemia followed by analyzing infarct volumes, which correlates to the area of cell death. This loss of function study showed neuronal injury in the knockout mice by the increase in the infarct volumes after either ischemia in comparison to the wild type. To identify the effects of the PrPC knockout on the mechanisms regulating cell death, Western blots were done to analyze the expression of proapoptotic and antiapoptotic proteins on mice with and without ischemia. It was shown that the deletion of PrPC led to a decrease in antiapoptotic and an increase in postapoptotic proteins. This and other experiments show that PrPC thereby mediates through several signal transduction pathways and aides in neuronal survival. This is probably why we see no severe detrimental effects in the cattle (i.e. the study conducted by Richt et al. (9) since PrPC functions only during neuronal injury. Since the cattle did not have neuronal injury we could not confirm whether PrPC has neuroprotective effects or not.
Future Studies
The above studies have paved the way for successful application of lentiviruses with RNA interference to suppress the PrPC gene in humans in the future and also indicated that suppression of the Prion protein permanently is a possible event. Loss of function techniques such as RNA interference will be helpful in gaining more insight into the physiological role of the Prion protein PrPC. Based on this evidence, the best way to lead to the suppression of CJD PrPC in vivo is to expand on the studies indicated. The human brain is much larger than that of mice, which were used as the model organism in many studies. It was confirmed that the mice with the high concentration of lentiviral vectors in the brain cells survived longer. Since the human brain is larger in size, to deliver high concentration of lentivectors throughout the human brain, multiple catheter’s or/and pharmacological opening of the blood brain barrier may be applied as suggested. After perfecting the procedures of the insertion of RNAi by lentivectors and overcoming the barriers during these procedures in a large brain sized mammal, preferably cow (a natural host of Prion disease), it can be certain that the suppression of Prion protein permanently in human beings will be carried out in the near future.
REFERENCES
(1) Kong Q. RNAi: a novel strategy for the treatment of prion diseases. The Journal of Clinical Investigation 2006; 116: 3101-3103.
(2) Alberts B, Johnson A, Lewis J, Raff M, Roberts K and Walter P. Molecular Biology of the Cell. 4th ed. New York: Garland Science; 2002. G:p. 31.
(3) Daude N, Marella M, Chabry J. Specific inhibition of pathological prion protein accumulation by small interfering RNAs. Journal of Cell Science 2003; 116: 2775-2779.
(4) Pfeifer A, Eigenbrod S, Al-Khadra S, Hofmann A, Mitteregger G, Moser M, Bertsch U, Kretzschmar H. Lentivector-mediated RNAi efficiently suppresses prion protein and prolongs survival of scrapie-infected mice. The Journal of Clinical Investigation 2006; 116: 3204-3210.
(5) Golding MC, Long CR, Carmell MA, Hannon GJ, Westhusin ME, Suppression of prion protein in livestock by RNA interference. PNAS 2006; 103: 5285-5290.
(6) Snove O, Rossi JJ. Expressing short harpin RNAs in vivo. Nat. Methods 2006; 3: 689-695.
(7) Xia XG, Zhou H, Xu Z. Promises and challenges in developing RNAi as a research tool and therapy for neurodegenerative diseases. Neurodegener. Dis. 2005; 2: 220-231.
(8) Sachdeva G, D’Costa J, Cho JE, Kachapati K, Choudhry V, Arya SK. Chimeric HIV-1 and HIV-2 Lentiviral Vectors With Added Safety Insurance. Journal of Medical Virology 2007; 79: 118-126.
(9) Richt JA, Kasinathan P, Hamir AN, Castilla J, Sathiyaseelan T, Vargas F, Sathiyaseelan J, Wu H, Matsushita H, Koster J, Kato S, Ishida I, Soto C, Robl JM, Kuroiwa Y. Production of cattle lacking prion protein. Nature Biotechnology 2007; 25: 132-138.
(10) Weise J, Sandau R, Schwarting S, Crome O, Wrede A, Schulz-Schaeffer W, Zerr I, Bähr M. Deletion of Cellular Prion Protein Results in Reduced Akt Activation, Enhanced Postischemic Caspase-3 Activation, and Exacerbation of Ischemic Brain Injury. Stroke 2006; 37: 1296-1300.